Home / The SN1 Mechanism
Substitution Reactions
The SN1 Mechanism
Last updated: April 26th, 2024 |
The SN1 Reaction Mechanism
- There are two important classes of nucleophilic substitution mechanisms – the SN1 and SN2 mechanisms (See article – Two Types of Substitution Reactions)
- The SN1 mechanism is distinct from the SN2 in three distinct ways.
- The reaction is fastest for tertiary alkyl halides and slowest for primary (and methyl) halides
- The rate law is unimolecular – it is only dependent on the concentration of substrate (i.e. alkyl halide) and not the nucleophile
- Alkyl halides with a chiral center at the “alpha-carbon” will give a product that provides a mixture of retention of configuration and inversion of configuration. [Note 2] Sometimes this is described as “racemization” .
- The best explanation for how this reaction works is that it begins with a (rate-determining) loss of a leaving group to give a carbocation, which can then undergo attack by a weak nucleophile at either face, resulting in the loss of stereochemistry.
- The SN1 reaction is sometimes accompanied by carbocation rearrangements. (See article – Substitution With Rearrangement)
Table of Contents
- Stereochemistry Of The SN1 Reaction: A Mixture of Retention and Inversion is Observed
- The Rate Law Of The SN1 Reaction Is First-Order Overall
- The Reaction Rate Increases With Substitution At Carbon (Tertiary >> Secondary > Primary)
- The Stepwise Reaction Mechanism of the SN1 Reaction
- Notes
- Quiz Yourself!
- (Advanced) References and Further Reading
1. Stereochemistry Of The SN1 Reaction: A Mixture of Retention and Inversion is Observed
If we start with an enantiomerically pure product, (that is, one enantiomer), these reactions tend to result in a mixture of products where the stereochemistry is the same as the starting material (retention) or opposite (inversion). In other words, some degree of racemization will take place (See post: What Is A Racemic Mixture?)
Compare this to the SN2, which always results in inversion of stereochemistry! Clearly something different must be going on here.
2. The Rate Law Of The SN1 Reaction Is First-Order Overall
We can also measure the rate law of these reactions. When we do so, we notice that the rate is only dependent on the concentration of the substrate, but not on the concentration of nucleophile.
Weird. Remember that the SN2 depends on both. Why might this reaction only depend on the concentration of substrate?
3. The Reaction Rate Increases With Substitution At Carbon
When we subtly change the types of substrates (e.g. alkyl halides) we use in these reactions, we find that tertiary substrates (for instance, t-butyl bromide) are considerably faster than secondary alkyl bromides, which are in turn faster than primary [Note 1]
Compare that to the case for SN2, where primary was faster than secondary and tertiary hardly reacted at all. Mysterious!
4. The Stepwise Reaction Mechanism of the SN1 Reaction
The best hypothesis we have for this reaction is a stepwise mechanism.
- In the first step, the leaving group leaves, forming a carbocation.
- In the second, a nucleophile attacks the carbocation, forming the new product.
This explains all of our observations nicely. First of all, the slow step should be formation of the (unstable) carbocation – which only depends on the substrate, not the nucleophile.
Furthermore, since the stability of carbocations depends tremendously on substitution pattern (tertiary carbocations are more stable than secondary, which are more stable than primary) this also conveniently explains the dependence of the reaction rate on substitution pattern (See post: Carbocation Stability)
Any factor which stabilizes the carbocation, increases the rate at which the leaving group can leave.
It also helps us understand the stereochemistry. Since the electrophile is flat, attack could occur from either face; which means that we obtain a mixture of retention and inversion products.
This is therefore called the SN1 mechanism – Substitution, Nucleophilic, Unimolecular – to contrast with the SN2 (Substitution, Nucleophilic, Bimolecular).
It all seems to work if you’ve got a good leaving group present (like a halogen). But what if you don’t have a good leaving group? In the next post we’ll talk about how to make a poor leaving group into a good one.
Next Post: The Conjugate Acid Is A Better Leaving Group
Notes
Related Articles
- The Conjugate Acid Is A Better Leaving Group
- The SN2 Mechanism
- 3 Factors That Stabilize Carbocations
- Comparing the SN1 and SN2 Reactions
- What makes a good leaving group?
- Substitution Practice – SN1 (MOC Membership)
- Two Types of Nucleophilic Substitution Reactions
- Identifying Where Substitution and Elimination Reactions Happen
Note 1. – the primary alkyl halide shown here is certainly reacting solely through an SN2 mechanism.
Note 2. Athough it’s often said that the SN1 proceeds with “racemization” of stereocenters, in practice a 50/50 split of stereocenters may not be obtained due to “ion pairing” effects.
In other words, the leaving group could leave, but not fully dissociate from the vicinity of the carbocation, which could block a nucleophile from attacking the electrophile from that face. For that reason it’s a little bit more correct to say that it proceeds with a “mixture of retention and inversion” rather than “racemization”.
Quiz Yourself!
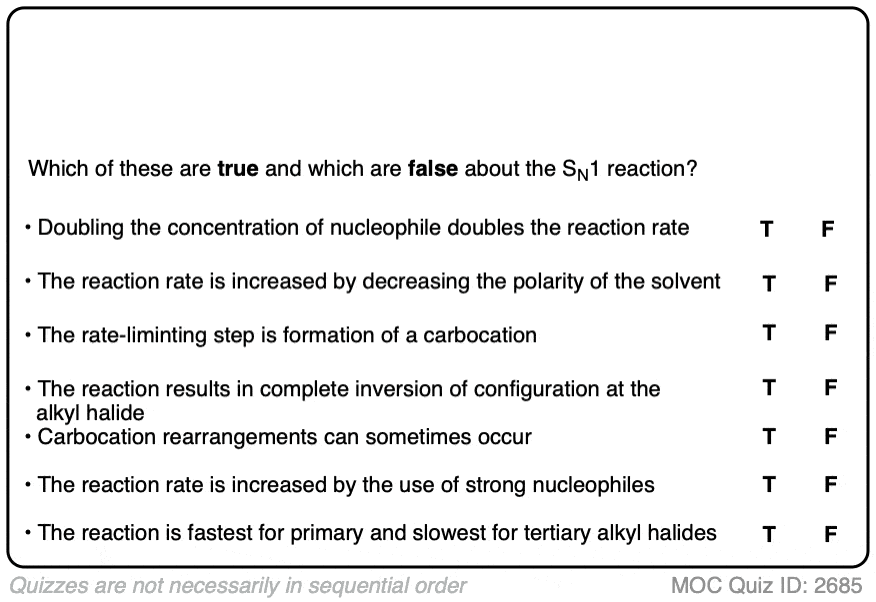
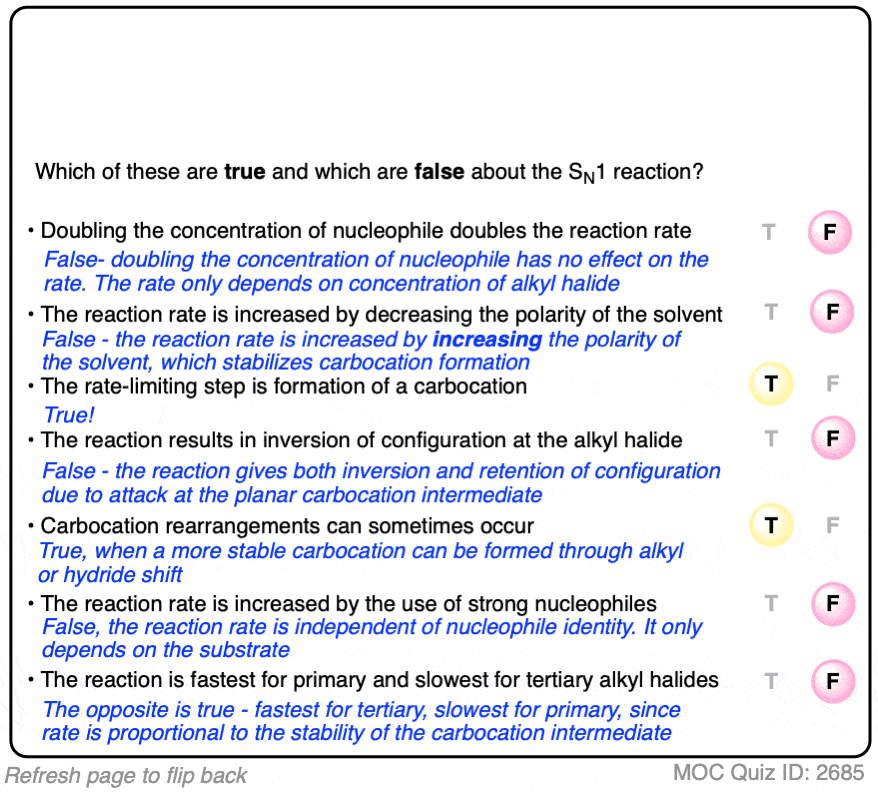
(Advanced) References and Further Reading
-
56. Mechanism of substitution at a saturated carbon atom. Part V. Hydrolysis of tert.-butyl chloride.
Edward D. Hughes.
J. Chem. Soc. 1935, 235
DOI: 10.1039/JR9350000255
Original study where the hydrolysis of t-butyl chloride was found to be first-order in alkyl halide and zero order in base, giving rise to the mechanism we now know as SN1. - Mechanism of substitution at a saturated carbon atom. Part IX. The rôle of the solvent in the first-order hydrolysis of alkyl halides
Leslie C. Bateman and Edward D. Hughes
J. Chem. Soc. 1937, 1187-1192
DOI: 10.1039/JR9370001187
In the hydrolysis of alkyl bromides by water in formic acid, the relative rates at 100° are MeBr 1.00, EtBr 1.71, iPrBr 44.7, and tBuBr ca. 10^8. - Reaction kinetics and the Walden inversion. Part I. Homogeneous hydrolysis and alcoholysis of β-n-octyl halides
Edward D. Hughes, Christopher K. Ingold and Standish Masterman
J. Chem. Soc. 1937, 1196-1201
DOI: 10.1039/JR9370001196 - Reaction kinetics and the Walden inversion. Part IV. Action of silver salts in hydroxylic solvents on β-n-octyl bromide and α-phenylethyl chloride
Edward D. Hughes, Christopher K. Ingold and Standish Masterman
J. Chem. Soc., 1937, 1236-1243
DOI: 10.1039/JR9370001236
These two papers examine reactions of 2-octyl halides in an attempt to see if pure SN1 or SN2 pathways on the same substrate can be favored simply by varying the reaction conditions. - The Correlation of Solvolysis Rates
Ernest Grunwald and S. Winstein
Journal of the American Chemical Society 1948, 70 (2), 846-854
DOI: 1021/ja01182a117
This is a very important paper, discussing the ‘Grunwald-Winstein equation’ for the first time. This equation is an extension of the Hammett equation, taking solvent effects (i.e. ‘ionizing power’) into consideration. - The Reactivity of Bridgehead Compounds of Adamantane
Paul von R. Schleyer and Robert D. Nicholas
Journal of the American Chemical Society 1961, 83 (12), 2700-2707
DOI: 1021/ja01473a024
Bridgehead carbocations are generally quite unstable since they cannot achieve the planar geometry necessary for good hyperconjugative stabilization. Somewhat surprisingly, in this paper it is found that the SN1 reaction of 1-bromoadamantane proceeds only about 1000 times slower than that of t-butyl bromide, albeit (of course) only with retention of configuration. - The Common Basis of Intramolecular Rearrangements. VI.1 Reactions of Neopentyl Iodide
Frank C. Whitmore, E. L. Wittle, and A. H. Popkin
Journal of the American Chemical Society 1939, 61 (6), 1586-1590
DOI: 1021/ja01875a073
An early paper demonstrating that SN1 reactions can be induced by reaction of an alkyl halide with silver salts. In this case, the neopentyl cation quickly rearranges to the significantly more stable t-amyl cation, and those products are obtained. - Mechanism of substitution at a saturated carbon atom. Part XXIX. The rôle of steric hindrance. (Section D) the mechanism of the reaction of neopentyl bromide with aqueous ethyl alcohol
I. Dostrovsky and E. D. Hughes
J. Chem. Soc., 1946, 166-169
DOI: 10.1039/JR9460000166
This is an example of an SN1 reaction with rearrangement. Neopentyl bromide in aqueous ethyl alcohol gives t-amyl alcohol (and t-amyl ethyl ether). - Mechanism of substitution at a saturated carbon atom. Part XXXV. Effect of temperature on the competition between unimolecular solvolytic and non-solvolytic substitutions of di-p-tolylmethyl chloride. Activation in the fast step of unimolecular non-solvolytic substitution
Audrey R. Hawdon, E. D. Hughes and C. K. Ingold
J. Chem. Soc., 1952, 2499-2503
DOI: 10.1039/JR9520002499
It is possible to run SN1 reactions in the presence of added nucleophile, such as in the hydrolysis of benzyl chlorides in the presence of added sodium azide. The separate rates of formation of the carbocation and production of the azide can thus be measured. - Methanolysis of Optically Active Hydrogen 2,4-Dimethylhexyl-4-phthalate
von E. Doering and Harold H. Zeiss
Journal of the American Chemical Society 1953, 75 (19), 4733-4738
DOI: 10.1021/ja01115a035
An early example of an SN1 reaction without full racemization. Prof. Doering proposes a mechanism in the paper, interesting read. - Quaternary stereocentres via an enantioconvergent catalytic SN1 reaction
Wendlandt, A.E., Vangal, P. & Jacobsen, E.N.
Nature 556, 447–451 (2018)
DOI: 1038/s41586-018-0042-1
This is a rare example of an asymmetric SN1 reaction – normally the SN1 reaction is taught as giving achiral products, but in this particular case it is possible to induce chirality because the carbocation is so highly stabilized (tertiary, benzylic, and propargylic).
00 General Chemistry Review
01 Bonding, Structure, and Resonance
- How Do We Know Methane (CH4) Is Tetrahedral?
- Hybrid Orbitals and Hybridization
- How To Determine Hybridization: A Shortcut
- Orbital Hybridization And Bond Strengths
- Sigma bonds come in six varieties: Pi bonds come in one
- A Key Skill: How to Calculate Formal Charge
- The Four Intermolecular Forces and How They Affect Boiling Points
- 3 Trends That Affect Boiling Points
- How To Use Electronegativity To Determine Electron Density (and why NOT to trust formal charge)
- Introduction to Resonance
- How To Use Curved Arrows To Interchange Resonance Forms
- Evaluating Resonance Forms (1) - The Rule of Least Charges
- How To Find The Best Resonance Structure By Applying Electronegativity
- Evaluating Resonance Structures With Negative Charges
- Evaluating Resonance Structures With Positive Charge
- Exploring Resonance: Pi-Donation
- Exploring Resonance: Pi-acceptors
- In Summary: Evaluating Resonance Structures
- Drawing Resonance Structures: 3 Common Mistakes To Avoid
- How to apply electronegativity and resonance to understand reactivity
- Bond Hybridization Practice
- Structure and Bonding Practice Quizzes
- Resonance Structures Practice
02 Acid Base Reactions
- Introduction to Acid-Base Reactions
- Acid Base Reactions In Organic Chemistry
- The Stronger The Acid, The Weaker The Conjugate Base
- Walkthrough of Acid-Base Reactions (3) - Acidity Trends
- Five Key Factors That Influence Acidity
- Acid-Base Reactions: Introducing Ka and pKa
- How to Use a pKa Table
- The pKa Table Is Your Friend
- A Handy Rule of Thumb for Acid-Base Reactions
- Acid Base Reactions Are Fast
- pKa Values Span 60 Orders Of Magnitude
- How Protonation and Deprotonation Affect Reactivity
- Acid Base Practice Problems
03 Alkanes and Nomenclature
- Meet the (Most Important) Functional Groups
- Condensed Formulas: Deciphering What the Brackets Mean
- Hidden Hydrogens, Hidden Lone Pairs, Hidden Counterions
- Don't Be Futyl, Learn The Butyls
- Primary, Secondary, Tertiary, Quaternary In Organic Chemistry
- Branching, and Its Affect On Melting and Boiling Points
- The Many, Many Ways of Drawing Butane
- Wedge And Dash Convention For Tetrahedral Carbon
- Common Mistakes in Organic Chemistry: Pentavalent Carbon
- Table of Functional Group Priorities for Nomenclature
- Summary Sheet - Alkane Nomenclature
- Organic Chemistry IUPAC Nomenclature Demystified With A Simple Puzzle Piece Approach
- Boiling Point Quizzes
- Organic Chemistry Nomenclature Quizzes
04 Conformations and Cycloalkanes
- Staggered vs Eclipsed Conformations of Ethane
- Conformational Isomers of Propane
- Newman Projection of Butane (and Gauche Conformation)
- Introduction to Cycloalkanes
- Geometric Isomers In Small Rings: Cis And Trans Cycloalkanes
- Calculation of Ring Strain In Cycloalkanes
- Cycloalkanes - Ring Strain In Cyclopropane And Cyclobutane
- Cyclohexane Conformations
- Cyclohexane Chair Conformation: An Aerial Tour
- How To Draw The Cyclohexane Chair Conformation
- The Cyclohexane Chair Flip
- The Cyclohexane Chair Flip - Energy Diagram
- Substituted Cyclohexanes - Axial vs Equatorial
- Ranking The Bulkiness Of Substituents On Cyclohexanes: "A-Values"
- Cyclohexane Chair Conformation Stability: Which One Is Lower Energy?
- Fused Rings - Cis-Decalin and Trans-Decalin
- Naming Bicyclic Compounds - Fused, Bridged, and Spiro
- Bredt's Rule (And Summary of Cycloalkanes)
- Newman Projection Practice
- Cycloalkanes Practice Problems
05 A Primer On Organic Reactions
- The Most Important Question To Ask When Learning a New Reaction
- Learning New Reactions: How Do The Electrons Move?
- The Third Most Important Question to Ask When Learning A New Reaction
- 7 Factors that stabilize negative charge in organic chemistry
- 7 Factors That Stabilize Positive Charge in Organic Chemistry
- Nucleophiles and Electrophiles
- Curved Arrows (for reactions)
- Curved Arrows (2): Initial Tails and Final Heads
- Nucleophilicity vs. Basicity
- The Three Classes of Nucleophiles
- What Makes A Good Nucleophile?
- What makes a good leaving group?
- 3 Factors That Stabilize Carbocations
- Equilibrium and Energy Relationships
- What's a Transition State?
- Hammond's Postulate
- Learning Organic Chemistry Reactions: A Checklist (PDF)
- Introduction to Free Radical Substitution Reactions
- Introduction to Oxidative Cleavage Reactions
06 Free Radical Reactions
- Bond Dissociation Energies = Homolytic Cleavage
- Free Radical Reactions
- 3 Factors That Stabilize Free Radicals
- What Factors Destabilize Free Radicals?
- Bond Strengths And Radical Stability
- Free Radical Initiation: Why Is "Light" Or "Heat" Required?
- Initiation, Propagation, Termination
- Monochlorination Products Of Propane, Pentane, And Other Alkanes
- Selectivity In Free Radical Reactions
- Selectivity in Free Radical Reactions: Bromination vs. Chlorination
- Halogenation At Tiffany's
- Allylic Bromination
- Bonus Topic: Allylic Rearrangements
- In Summary: Free Radicals
- Synthesis (2) - Reactions of Alkanes
- Free Radicals Practice Quizzes
07 Stereochemistry and Chirality
- Types of Isomers: Constitutional Isomers, Stereoisomers, Enantiomers, and Diastereomers
- How To Draw The Enantiomer Of A Chiral Molecule
- How To Draw A Bond Rotation
- Introduction to Assigning (R) and (S): The Cahn-Ingold-Prelog Rules
- Assigning Cahn-Ingold-Prelog (CIP) Priorities (2) - The Method of Dots
- Enantiomers vs Diastereomers vs The Same? Two Methods For Solving Problems
- Assigning R/S To Newman Projections (And Converting Newman To Line Diagrams)
- How To Determine R and S Configurations On A Fischer Projection
- The Meso Trap
- Optical Rotation, Optical Activity, and Specific Rotation
- Optical Purity and Enantiomeric Excess
- What's a Racemic Mixture?
- Chiral Allenes And Chiral Axes
- Stereochemistry Practice Problems and Quizzes
08 Substitution Reactions
- Nucleophilic Substitution Reactions - Introduction
- Two Types of Nucleophilic Substitution Reactions
- The SN2 Mechanism
- Why the SN2 Reaction Is Powerful
- The SN1 Mechanism
- The Conjugate Acid Is A Better Leaving Group
- Comparing the SN1 and SN2 Reactions
- Polar Protic? Polar Aprotic? Nonpolar? All About Solvents
- Steric Hindrance is Like a Fat Goalie
- Common Blind Spot: Intramolecular Reactions
- Substitution Practice - SN1
- Substitution Practice - SN2
09 Elimination Reactions
- Elimination Reactions (1): Introduction And The Key Pattern
- Elimination Reactions (2): The Zaitsev Rule
- Elimination Reactions Are Favored By Heat
- Two Elimination Reaction Patterns
- The E1 Reaction
- The E2 Mechanism
- E1 vs E2: Comparing the E1 and E2 Reactions
- Antiperiplanar Relationships: The E2 Reaction and Cyclohexane Rings
- Bulky Bases in Elimination Reactions
- Comparing the E1 vs SN1 Reactions
- Elimination (E1) Reactions With Rearrangements
- E1cB - Elimination (Unimolecular) Conjugate Base
- Elimination (E1) Practice Problems And Solutions
- Elimination (E2) Practice Problems and Solutions
10 Rearrangements
11 SN1/SN2/E1/E2 Decision
- Identifying Where Substitution and Elimination Reactions Happen
- Deciding SN1/SN2/E1/E2 (1) - The Substrate
- Deciding SN1/SN2/E1/E2 (2) - The Nucleophile/Base
- SN1 vs E1 and SN2 vs E2 : The Temperature
- Deciding SN1/SN2/E1/E2 - The Solvent
- Wrapup: The Key Factors For Determining SN1/SN2/E1/E2
- Alkyl Halide Reaction Map And Summary
- SN1 SN2 E1 E2 Practice Problems
12 Alkene Reactions
- E and Z Notation For Alkenes (+ Cis/Trans)
- Alkene Stability
- Alkene Addition Reactions: "Regioselectivity" and "Stereoselectivity" (Syn/Anti)
- Stereoselective and Stereospecific Reactions
- Hydrohalogenation of Alkenes and Markovnikov's Rule
- Hydration of Alkenes With Aqueous Acid
- Rearrangements in Alkene Addition Reactions
- Halogenation of Alkenes and Halohydrin Formation
- Oxymercuration Demercuration of Alkenes
- Hydroboration Oxidation of Alkenes
- m-CPBA (meta-chloroperoxybenzoic acid)
- OsO4 (Osmium Tetroxide) for Dihydroxylation of Alkenes
- Palladium on Carbon (Pd/C) for Catalytic Hydrogenation of Alkenes
- Cyclopropanation of Alkenes
- A Fourth Alkene Addition Pattern - Free Radical Addition
- Alkene Reactions: Ozonolysis
- Summary: Three Key Families Of Alkene Reaction Mechanisms
- Synthesis (4) - Alkene Reaction Map, Including Alkyl Halide Reactions
- Alkene Reactions Practice Problems
13 Alkyne Reactions
- Acetylides from Alkynes, And Substitution Reactions of Acetylides
- Partial Reduction of Alkynes With Lindlar's Catalyst
- Partial Reduction of Alkynes With Na/NH3 To Obtain Trans Alkenes
- Alkyne Hydroboration With "R2BH"
- Hydration and Oxymercuration of Alkynes
- Hydrohalogenation of Alkynes
- Alkyne Halogenation: Bromination, Chlorination, and Iodination of Alkynes
- Alkyne Reactions - The "Concerted" Pathway
- Alkenes To Alkynes Via Halogenation And Elimination Reactions
- Alkynes Are A Blank Canvas
- Synthesis (5) - Reactions of Alkynes
- Alkyne Reactions Practice Problems With Answers
14 Alcohols, Epoxides and Ethers
- Alcohols - Nomenclature and Properties
- Alcohols Can Act As Acids Or Bases (And Why It Matters)
- Alcohols - Acidity and Basicity
- The Williamson Ether Synthesis
- Ethers From Alkenes, Tertiary Alkyl Halides and Alkoxymercuration
- Alcohols To Ethers via Acid Catalysis
- Cleavage Of Ethers With Acid
- Epoxides - The Outlier Of The Ether Family
- Opening of Epoxides With Acid
- Epoxide Ring Opening With Base
- Making Alkyl Halides From Alcohols
- Tosylates And Mesylates
- PBr3 and SOCl2
- Elimination Reactions of Alcohols
- Elimination of Alcohols To Alkenes With POCl3
- Alcohol Oxidation: "Strong" and "Weak" Oxidants
- Demystifying The Mechanisms of Alcohol Oxidations
- Protecting Groups For Alcohols
- Thiols And Thioethers
- Calculating the oxidation state of a carbon
- Oxidation and Reduction in Organic Chemistry
- Oxidation Ladders
- SOCl2 Mechanism For Alcohols To Alkyl Halides: SN2 versus SNi
- Alcohol Reactions Roadmap (PDF)
- Alcohol Reaction Practice Problems
- Epoxide Reaction Quizzes
- Oxidation and Reduction Practice Quizzes
15 Organometallics
- What's An Organometallic?
- Formation of Grignard and Organolithium Reagents
- Organometallics Are Strong Bases
- Reactions of Grignard Reagents
- Protecting Groups In Grignard Reactions
- Synthesis Problems Involving Grignard Reagents
- Grignard Reactions And Synthesis (2)
- Organocuprates (Gilman Reagents): How They're Made
- Gilman Reagents (Organocuprates): What They're Used For
- The Heck, Suzuki, and Olefin Metathesis Reactions (And Why They Don't Belong In Most Introductory Organic Chemistry Courses)
- Reaction Map: Reactions of Organometallics
- Grignard Practice Problems
16 Spectroscopy
- Degrees of Unsaturation (or IHD, Index of Hydrogen Deficiency)
- Conjugation And Color (+ How Bleach Works)
- Introduction To UV-Vis Spectroscopy
- UV-Vis Spectroscopy: Absorbance of Carbonyls
- UV-Vis Spectroscopy: Practice Questions
- Bond Vibrations, Infrared Spectroscopy, and the "Ball and Spring" Model
- Infrared Spectroscopy: A Quick Primer On Interpreting Spectra
- IR Spectroscopy: 4 Practice Problems
- 1H NMR: How Many Signals?
- Homotopic, Enantiotopic, Diastereotopic
- Diastereotopic Protons in 1H NMR Spectroscopy: Examples
- C13 NMR - How Many Signals
- Liquid Gold: Pheromones In Doe Urine
- Natural Product Isolation (1) - Extraction
- Natural Product Isolation (2) - Purification Techniques, An Overview
- Structure Determination Case Study: Deer Tarsal Gland Pheromone
17 Dienes and MO Theory
- What To Expect In Organic Chemistry 2
- Are these molecules conjugated?
- Conjugation And Resonance In Organic Chemistry
- Bonding And Antibonding Pi Orbitals
- Molecular Orbitals of The Allyl Cation, Allyl Radical, and Allyl Anion
- Pi Molecular Orbitals of Butadiene
- Reactions of Dienes: 1,2 and 1,4 Addition
- Thermodynamic and Kinetic Products
- More On 1,2 and 1,4 Additions To Dienes
- s-cis and s-trans
- The Diels-Alder Reaction
- Cyclic Dienes and Dienophiles in the Diels-Alder Reaction
- Stereochemistry of the Diels-Alder Reaction
- Exo vs Endo Products In The Diels Alder: How To Tell Them Apart
- HOMO and LUMO In the Diels Alder Reaction
- Why Are Endo vs Exo Products Favored in the Diels-Alder Reaction?
- Diels-Alder Reaction: Kinetic and Thermodynamic Control
- The Retro Diels-Alder Reaction
- The Intramolecular Diels Alder Reaction
- Regiochemistry In The Diels-Alder Reaction
- The Cope and Claisen Rearrangements
- Electrocyclic Reactions
- Electrocyclic Ring Opening And Closure (2) - Six (or Eight) Pi Electrons
- Diels Alder Practice Problems
- Molecular Orbital Theory Practice
18 Aromaticity
- Introduction To Aromaticity
- Rules For Aromaticity
- Huckel's Rule: What Does 4n+2 Mean?
- Aromatic, Non-Aromatic, or Antiaromatic? Some Practice Problems
- Antiaromatic Compounds and Antiaromaticity
- The Pi Molecular Orbitals of Benzene
- The Pi Molecular Orbitals of Cyclobutadiene
- Frost Circles
- Aromaticity Practice Quizzes
19 Reactions of Aromatic Molecules
- Electrophilic Aromatic Substitution: Introduction
- Activating and Deactivating Groups In Electrophilic Aromatic Substitution
- Electrophilic Aromatic Substitution - The Mechanism
- Ortho-, Para- and Meta- Directors in Electrophilic Aromatic Substitution
- Understanding Ortho, Para, and Meta Directors
- Why are halogens ortho- para- directors?
- Disubstituted Benzenes: The Strongest Electron-Donor "Wins"
- Electrophilic Aromatic Substitutions (1) - Halogenation of Benzene
- Electrophilic Aromatic Substitutions (2) - Nitration and Sulfonation
- EAS Reactions (3) - Friedel-Crafts Acylation and Friedel-Crafts Alkylation
- Intramolecular Friedel-Crafts Reactions
- Nucleophilic Aromatic Substitution (NAS)
- Nucleophilic Aromatic Substitution (2) - The Benzyne Mechanism
- Reactions on the "Benzylic" Carbon: Bromination And Oxidation
- The Wolff-Kishner, Clemmensen, And Other Carbonyl Reductions
- More Reactions on the Aromatic Sidechain: Reduction of Nitro Groups and the Baeyer Villiger
- Aromatic Synthesis (1) - "Order Of Operations"
- Synthesis of Benzene Derivatives (2) - Polarity Reversal
- Aromatic Synthesis (3) - Sulfonyl Blocking Groups
- Birch Reduction
- Synthesis (7): Reaction Map of Benzene and Related Aromatic Compounds
- Aromatic Reactions and Synthesis Practice
- Electrophilic Aromatic Substitution Practice Problems
20 Aldehydes and Ketones
- What's The Alpha Carbon In Carbonyl Compounds?
- Nucleophilic Addition To Carbonyls
- Aldehydes and Ketones: 14 Reactions With The Same Mechanism
- Sodium Borohydride (NaBH4) Reduction of Aldehydes and Ketones
- Grignard Reagents For Addition To Aldehydes and Ketones
- Wittig Reaction
- Hydrates, Hemiacetals, and Acetals
- Imines - Properties, Formation, Reactions, and Mechanisms
- All About Enamines
- Breaking Down Carbonyl Reaction Mechanisms: Reactions of Anionic Nucleophiles (Part 2)
- Aldehydes Ketones Reaction Practice
21 Carboxylic Acid Derivatives
- Nucleophilic Acyl Substitution (With Negatively Charged Nucleophiles)
- Addition-Elimination Mechanisms With Neutral Nucleophiles (Including Acid Catalysis)
- Basic Hydrolysis of Esters - Saponification
- Transesterification
- Proton Transfer
- Fischer Esterification - Carboxylic Acid to Ester Under Acidic Conditions
- Lithium Aluminum Hydride (LiAlH4) For Reduction of Carboxylic Acid Derivatives
- LiAlH[Ot-Bu]3 For The Reduction of Acid Halides To Aldehydes
- Di-isobutyl Aluminum Hydride (DIBAL) For The Partial Reduction of Esters and Nitriles
- Amide Hydrolysis
- Thionyl Chloride (SOCl2)
- Diazomethane (CH2N2)
- Carbonyl Chemistry: Learn Six Mechanisms For the Price Of One
- Making Music With Mechanisms (PADPED)
- Carboxylic Acid Derivatives Practice Questions
22 Enols and Enolates
- Keto-Enol Tautomerism
- Enolates - Formation, Stability, and Simple Reactions
- Kinetic Versus Thermodynamic Enolates
- Aldol Addition and Condensation Reactions
- Reactions of Enols - Acid-Catalyzed Aldol, Halogenation, and Mannich Reactions
- Claisen Condensation and Dieckmann Condensation
- Decarboxylation
- The Malonic Ester and Acetoacetic Ester Synthesis
- The Michael Addition Reaction and Conjugate Addition
- The Robinson Annulation
- Haloform Reaction
- The Hell–Volhard–Zelinsky Reaction
- Enols and Enolates Practice Quizzes
23 Amines
- The Amide Functional Group: Properties, Synthesis, and Nomenclature
- Basicity of Amines And pKaH
- 5 Key Basicity Trends of Amines
- The Mesomeric Effect And Aromatic Amines
- Nucleophilicity of Amines
- Alkylation of Amines (Sucks!)
- Reductive Amination
- The Gabriel Synthesis
- Some Reactions of Azides
- The Hofmann Elimination
- The Hofmann and Curtius Rearrangements
- The Cope Elimination
- Protecting Groups for Amines - Carbamates
- The Strecker Synthesis of Amino Acids
- Introduction to Peptide Synthesis
- Reactions of Diazonium Salts: Sandmeyer and Related Reactions
- Amine Practice Questions
24 Carbohydrates
- D and L Notation For Sugars
- Pyranoses and Furanoses: Ring-Chain Tautomerism In Sugars
- What is Mutarotation?
- Reducing Sugars
- The Big Damn Post Of Carbohydrate-Related Chemistry Definitions
- The Haworth Projection
- Converting a Fischer Projection To A Haworth (And Vice Versa)
- Reactions of Sugars: Glycosylation and Protection
- The Ruff Degradation and Kiliani-Fischer Synthesis
- Isoelectric Points of Amino Acids (and How To Calculate Them)
- Carbohydrates Practice
- Amino Acid Quizzes
25 Fun and Miscellaneous
- A Gallery of Some Interesting Molecules From Nature
- Screw Organic Chemistry, I'm Just Going To Write About Cats
- On Cats, Part 1: Conformations and Configurations
- On Cats, Part 2: Cat Line Diagrams
- On Cats, Part 4: Enantiocats
- On Cats, Part 6: Stereocenters
- Organic Chemistry Is Shit
- The Organic Chemistry Behind "The Pill"
- Maybe they should call them, "Formal Wins" ?
- Why Do Organic Chemists Use Kilocalories?
- The Principle of Least Effort
- Organic Chemistry GIFS - Resonance Forms
- Reproducibility In Organic Chemistry
- What Holds The Nucleus Together?
- How Reactions Are Like Music
- Organic Chemistry and the New MCAT
26 Organic Chemistry Tips and Tricks
- Common Mistakes: Formal Charges Can Mislead
- Partial Charges Give Clues About Electron Flow
- Draw The Ugly Version First
- Organic Chemistry Study Tips: Learn the Trends
- The 8 Types of Arrows In Organic Chemistry, Explained
- Top 10 Skills To Master Before An Organic Chemistry 2 Final
- Common Mistakes with Carbonyls: Carboxylic Acids... Are Acids!
- Planning Organic Synthesis With "Reaction Maps"
- Alkene Addition Pattern #1: The "Carbocation Pathway"
- Alkene Addition Pattern #2: The "Three-Membered Ring" Pathway
- Alkene Addition Pattern #3: The "Concerted" Pathway
- Number Your Carbons!
- The 4 Major Classes of Reactions in Org 1
- How (and why) electrons flow
- Grossman's Rule
- Three Exam Tips
- A 3-Step Method For Thinking Through Synthesis Problems
- Putting It Together
- Putting Diels-Alder Products in Perspective
- The Ups and Downs of Cyclohexanes
- The Most Annoying Exceptions in Org 1 (Part 1)
- The Most Annoying Exceptions in Org 1 (Part 2)
- The Marriage May Be Bad, But the Divorce Still Costs Money
- 9 Nomenclature Conventions To Know
- Nucleophile attacks Electrophile
27 Case Studies of Successful O-Chem Students
- Success Stories: How Corina Got The The "Hard" Professor - And Got An A+ Anyway
- How Helena Aced Organic Chemistry
- From a "Drop" To B+ in Org 2 – How A Hard Working Student Turned It Around
- How Serge Aced Organic Chemistry
- Success Stories: How Zach Aced Organic Chemistry 1
- Success Stories: How Kari Went From C– to B+
- How Esther Bounced Back From a "C" To Get A's In Organic Chemistry 1 And 2
- How Tyrell Got The Highest Grade In Her Organic Chemistry Course
- This Is Why Students Use Flashcards
- Success Stories: How Stu Aced Organic Chemistry
- How John Pulled Up His Organic Chemistry Exam Grades
- Success Stories: How Nathan Aced Organic Chemistry (Without It Taking Over His Life)
- How Chris Aced Org 1 and Org 2
- Interview: How Jay Got an A+ In Organic Chemistry
- How to Do Well in Organic Chemistry: One Student's Advice
- "America's Top TA" Shares His Secrets For Teaching O-Chem
- "Organic Chemistry Is Like..." - A Few Metaphors
- How To Do Well In Organic Chemistry: Advice From A Tutor
- Guest post: "I went from being afraid of tests to actually looking forward to them".
If after nucleophilic attack, the carbon is not a chiral centre, then wont it just give us a retained configuration product?
For something like t-butyl bromide going to t-butyl alcohol, there is no possibility of forming stereoisomers, so the question of retention or inversion becomes a non-issue.
I’m still learning about electrophilic sub/elim reactions, and your website is a blessing! I’ve got one question regarding the fast method to determine a reaction is SN1 or SN2. Let’s say that Br- is the leaving group. It’s said that if a nucleophilic substitution reaction has an acid (in this case HBr) as its product, then it’s a SN1 reaction. On the other hand, if a nucleophilic substitution reaction has a anion (in this case Br-), then it’s a SN2 reaction. I’m sure this method is useful and heuristic, but whether it be SN1 or SN2, shouldn’t the leaving group Br- not able to form HBr (as it’s a weak conjugate base)? I assume you wrote H3O+ Br- to show this, but I might be wrong. Would you please give some clarification on this? Thank you!
You’ll likely only ever see HBr if you are performing substitution reactions on alcohols. The purpose is to turn the poor leaving group OH into the good leaving group H2O, and then, depending on whether the substrate is primary (SN2) tertiary (SN1) or secondary (ask your instructor, answers vary!) you will obtain the resulting substitution product.
Hey James, I recently came across this paper: http://dx.doi.org/10.1021/ed086p519
Does this mean that the SN1 mechanism is not applicable for secondary alkyl halides at all? If so, please make a post explaining why (I’m still a student, and don’t really understand the paper)
That is a very interesting paper.
I would not say that the SN1 doesn’t apply to secondary alkyl halides *at all* but let’s just say they are much less important than they appear to be.
Let’s just say that the whole teaching of SN1/SN2/E1/E2 is pretty muddled, and has been this way for quite some time. If you take introductory organic you would come away thinking that organic chemists spend a great deal of their time trying to plan out SN1/Sn2/e1/e2 reactions whereas in reality the ratio of published SN2 and E2 reactions vs SN1 and E1 reactions is at least 100:1. Even then the SN2 is best done on primary substrates to avoid side reactions.
It’s a mess. I’m glad someone has gone back and looked through the original Ingold/Hughes papers, because they are the basis for a lot of what is taught.
Can kinetics provide all the necessary details about nucleophilic substitution reactions?
I’m not sure what details you mean by “all necessary details”, but even a limited reading of that would neglect issues like stereochemistry, etc. So the answer would be no.
Awesome and helpful content- specific typo is under #3. types of substrates***
Thanks!
Not seeing it. Anyone?
Thanks for the very helpful site!
I have a question about the first step in an SN1 reaction – the leaving group leaves. What causes the leaving group to want to leave? Is this action set in motion by heat, light or some other energy source?
Thanks for the help.
It’s helpful to think of bonds as balls and springs. At any given moment the bond between the carbon and the leaving group is oscillating like two balls connected by a spring, and the bond length is alternating between being shorter than normal and longer than normal. The average is the “bond length”.
Now imagine the energy as that bond extends to its longest length. The carbon will have a partial positive charge (delta plus) and the leaving group will have a partial negative charge (delta minus). If the carbon is able to stabilize positive charge very well (e.g. a tertiary carbocation) and the leaving group is able to stabilize negative charge very well (e.g. a weak base) then it’s not hard to imagine the bond length getting longer and longer until it goes beyond the length of what we’d consider a normal bond and can be considered a carbocation in the vicinity of a leaving group. If a nucleophilic solvent is around (like CH3OH) it may then “trap” that free carbocation, resulting in a substitution reaction.
conversely if we have a situation like CH3CH2OH, where we have a bad leaving group (OH) connected to a carbon poorly able to stabilize positive charge (primary) then the energetic barrier for that bond length getting long enough to lead to dissociation is just too high.
In sn1 rexn most usually racemic mixture is formed in product but it isnot 100% racemized,why?
The reason is that the resulting carbocation and the leaving group can form what is known as a “tight ion pair”. In a tight ion pair the leaving group has not completely dissociated, and this will block the face of the carbocation that was connected to the leaving group.
In order for full racemization to occur there needs to be complete dissociation such that either face of the carbocation can be attacked with equal probability.
Would you please write a textbook – I would gladly buy it!
No plans as yet, but thanks for the vote of confidence!
I can’t tell you how brilliantly every topic is explained. This is great. Your analogies and phrasing are the only way I understand organic chem. Thanks a ton
Glad to hear it Anisha!
I got it. thank you
In the paragraph under “final note”, I believe “In other words, the leaving group could leave, but not fully dissociate from the vicinity of the carbocation, which could block a nucleophile from attacking the nucleophile from that face” should read “block a nucleophile from attacking the electrophile” instead.Also,In the paragraph under “So what could be going on here?”, I believe “Since the nucleophile is flat, attack could occur from either face; which means that we obtain a mixture of retention and inversion products” should read “Since the electrophile is flat” instead.
sir plz tell me the percentage of inverted and retented prodct in ion pair mechanism…???
It would depend on a variety of factors, such as temperature, solvent, the identity of the leaving group and the substrate. Highly variable!
EXTREMELY helpful! The content is very straightforward and understandable! MUCH appreciated! This website will be used for the whole semester!!
yes … very helpful but organic chemistry is very intrusting chemistry than other branches of chemistry <3
You’ve created an awesome website here. I haven’t even used your reaction guide much yet, but I still like being a member simply because I feel like you totally deserve my money for all the help I’m getting from the blog. (It’s taught me more in a few days than my book has all year!)
Anyway, just wanted to point out that on your starred note at the bottom, I think you meant that the primary alkyl halide is certainly reacting through an SN2 mechanism (not an SN1).
Yes, you’re right. Thanks for the catch – and glad you find it useful!
Tremendously useful site, your efforts are highly appreciative, Kindly correct the spelling of Carbocation in the fourth line after mechanism.
thank you.